Abstract
Background: Sensory processing sensitivity (SPS) is a biologically based temperament trait associated with enhanced awareness and responsivity to environmental and social stimuli. Individuals with high SPS are more affected by their environments, which may result in overarousal, cognitive depletion, and fatigue. Method: We examined individual differences in resting-state (rs) brain connectivity (using functional MRI) as a function of SPS among a group of adults (M age = 66.13 ± 11.44 years) immediately after they completed a social affective “empathy” task. SPS was measured with the Highly Sensitive Person (HSP) Scale and correlated with rs brain connectivity. Results: Results showed enhanced rs brain connectivity within the ventral attention, dorsal attention, and limbic networks as a function of greater SPS. Region of interest analyses showed increased rs brain connectivity between the hippocampus and the precuneus (implicated in episodic memory); while weaker connectivity was shown between the amygdala and the periaqueductal gray (important for anxiety), and the hippocampus and insula (implicated in habitual cognitive processing). Conclusions: The present study showed that SPS is associated with rs brain connectivity implicated in attentional control, consolidation of memory, physiological homeostasis, and deliberative cognition. These results support theories proposing “depth of processing” as a central feature of SPS and highlight the neural processes underlying this cardinal feature of the trait.
Introduction
Sensory processing sensitivity (SPS), sometimes also referred to as environmental sensitivity (ES) [1], is a biologically based temperament trait characterized by deeper cognitive processing of stimuli, attention to subtleties, emotional reactivity, ease of overstimulation, and a greater awareness of subtleties in the environmental, including others affective states [2, 3]. Individual differences in SPS are determined by both biological factors and environmental influences, including early-childhood and developmental circumstances [4]. SPS has been associated with various candidate genes [5‒8], and twin studies have shown that its heritability is about 0.47 [9], similar to other temperament traits thought to be genetically based (the “missing” genetic association being best explained by the complexity of the inheritance of traits [10]).
The differences and similarities among other temperament traits and SPS will not be explored here beyond noting that several other theories, such as Rothbart’s concept of effortful control, have been associated with empathy in similar ways [11]. For example, pausing before acting allows time to notice the feelings of others and subtleties in the environment, while impulsivity [12] and strong behavioral activation system activity would interfere [13]. An observer-rated behavioral measure of SPS in young children also suggests a behavioral link between empathy and SPS, by showing that highly sensitive children, among other predicted behaviors, were more cautious yet more cooperative with an unfamiliar, friendly adult figure [14]. More recent developments in temperament theory can also be related to SPS, and specifically to careful processing. For example, Trofimova and Robbins’ [15] concept of “sensitivity to probabilities” as a type of arousal related to specific neurochemical activities shows similarities to SPS and may help explain the type of overarousal experienced by those with high SPS when overstimulated. Space does not permit a full discussion of the relationship between SPS (or environmental sensitivity) to other temperament conceptualizations, but it constitutes more than half of a recent review of SPS [3], in which SPS is discussed in light of central temperament theories [16‒21].
The idea of sensitivity to the environment as a temperament trait is now accepted throughout biology [3]. Similar terms such as responsivity, plasticity, arousability, and flexibility have become common in biology, given that individual differences in responsivity to the environment and other stimuli [15, 22‒25] are now commonly discussed and have been observed in over 100 nonhuman species, including fish [26], birds [27], rodents [28], and primates [29]. These differences in responsivity seem to occur in only a minority of individuals within any species due to negative frequency-dependent selection; that is, it provides an advantage in reproductive success only when not too many others have that trait. In humans, high SPS occurs in approximately 20–30% of the population [30‒32], and brain activation studies indicate considerable evidence of this more thorough processing and learning from experiences, especially when an experience has emotional significance [33].
An additional feature of SPS in humans relevant to this study is that according to theories of differential susceptibility [4, 34] and biological sensitivity to context [35], individuals with high SPS are more affected by their environments, both for better and for worse. Specifically, indicators of health, social competence, and so forth are enhanced if the high SPS individual has been reared in a good environment [24]. However, in response to poor-quality environments and to current stress, those with high SPS report greater fatigue, anxiety, depression, neuroticism (N), and diminished life and work satisfaction (for review, see [36]). Interestingly, many of the deficiencies associated with high SPS are largely moderated by negative childhoods [37]. Also, in overstimulating or emotionally demanding environments, those higher in SPS show increased levels of arousal, higher stress, lower attention, and cognitive depletion [1‒3, 38]. This susceptibility to their environment also makes those high in SPS more responsive to positive interventions. For example, in 2 studies, adolescents with high (vs. low) SPS showed significantly greater sustained decreases in externalizing behavior and depression in response to cognitive behavioral interventions [39, 40]. Thus, individuals with high SPS may especially benefit from rest and restorative activities. Indeed, high SPS is associated with needing more downtime to recover from busy days and overstimulation in the workplace [41]. Also, several items from the widely used Highly Sensitive Person (HSP) Scale [42] (which is based on extensive interviews with those high in SPS) assess “needing to withdraw into bed or into a darkened room during busy days,” “getting rattled when having a lot to do in a short amount of time,” and “getting annoyed when having to do too many things at once.” Rest may also be critical for engaging in depth of processing, another cardinal feature of the SPS trait [2, 42].
Present Study
In sum, SPS is associated with greater responsivity to the environment and social stimuli, both for better and for worse. Thus, in response to challenge, overstimulation, and cognitive exertion, those high in SPS experience overarousal, cognitive depletion, and fatigue. As such, highly sensitive persons may especially benefit from rest and restorative activities after challenging or stressful events [3]. However, no study has examined the brain at “rest” as a function of SPS to understand what processes may be taking place during rest. Thus, in the present study we examined individual differences in resting-state (rs) brain connectivity (using fMRI) associated with SPS among a group of adults immediately after they completed a social/affective “empathy” task used in a previous study [2]. Similar concepts have been included in temperament models, including sensitivity, empathy, and sensation-seeking [15, 16, 43]. We hypothesized that subsequent to an emotionally evocative empathy task, when given the opportunity to rest, higher SPS would be associated with stronger rs brain connectivity, reflecting depth of processing, attention, memory, and emotional processing. In addition, we examined regions of interest (ROIs) from previous task-based imaging studies of SPS (for review, see Acevedo et al. [33]) for replicated effects across active- and rs brain activity.
It is important to note that this sample was somewhat unique in that study participants were caregivers of a spouse experiencing mild cognitive impairment (MCI). Also, our participants completed a 6-week mind-body intervention prior to brain scanning. Mind-body practices have been shown to be beneficial in reducing stress, depression, and mood issues, as well as improving resiliency, memory, and executive functioning [44‒48]. Thus, we predicted that this group might reflect cognitive processing and homeostatic effects to an even greater extent as a function of high sensitivity given prior studies showing SPS’ association with responsiveness to interventions [39, 40].
Method
Participants
Participants were recruited via flyers, Internet ads, word of mouth, and at lectures in the Santa Barbara, CA community. Recruitment criteria were as follows: individuals ages 30–80, living with or married to a partner experiencing memory issues, able to commit to a 6-week (90-min/week) yoga class on campus, overall good health, and no fMRI contraindications (e.g., use of antidepressants, metal in or on the body, and pregnancy). All participants provided informed consent and received payment for their participation according to IRB procedures at the University of California, Santa Barbara (UCSB). No participant included in the study reported a history of any disorder (e.g., anxiety, personality disorders, or social phobia) or use of medications that might bias responses.
Participants were 15 (9 females and 6 males) healthy, right-handed individuals, ages 40–78 (M = 66.13 ± 11.44 years), and married or living with a partner (M = 29.04 ± 17.50 years, range 5.00–50.00 years). All subjects had completed college, including 3 with master’s degrees and 3 with doctoral degrees. Seven were employed (full-time, part-time, or self-employed), one was a homemaker, and 7 were retired. The mean annual household income of the sample was $107,700 USD (±$50,068, range $35,000–$220,000), and the ethnic/racial composition included 14 Caucasian individuals and one Hispanic-American.
Self-Report Measures
Participants completed a battery of questionnaires, including the 11-item version of the HSP Scale [42] measure of SPS and the 2-item N measure from the Big Five Inventory [49]. All items were assessed on a 7-item Likert scale. The original 27-item HSP Scale is unidimensional with alphas of 0.65 to 0.85 across numerous samples [50‒52]. The 11 items of the short-version HSP Scale were selected based on their high correlations with the overall scale score [53]. In the present study, the 11-item HSP Scale Cronbach’s α was 0.93, which is relatively similar to Cronbach’s alphas of previous studies using the 11-item (α ∼ 0.82) and the 27-item HSP Scale (α ∼ 0.85).
N, also known as negative affectivity, was measured with a 2-item measure, as in previous studies of SPS [2, 54]. The 2 items ask participants to describe themselves on a scale from 1 (strongly disagree) to 7 (strongly agree), as generally being (1) anxious, easily upset, and (2) calm, emotionally stable (reverse scored). N was measured as a control for negative affectivity which, if not controlled for, distorts HSP Scale scores because negative affectivity is moderately correlated with SPS [55]. Mainly, the high correlation between SPS and N is due to the negative tone of many of the HSP Scale items measuring response to overstimulation [3].
Procedures
Upon completing the study screening and prior to starting the 6-week mind-body program, participants completed a packet of baseline questionnaires. During the 6-week intervention, participants were asked to record their daily at-home meditation activity. After completing the 6-week mind-body program, participants completed postintervention questionnaires and were scheduled for the fMRI scan. The day prior to scanning, subjects were given a verbal description of the fMRI procedures and were screened again for any fMRI contraindications.
fMRI Social Affective Task
The fMRI scanning task was conducted in 2 parts. Part one consisted of an “empathy” task used in prior research, including a study on the neural basis of SPS [2, 56]. The empathy protocol asked participants to read descriptions of an emotional (happy or sad) or neutral event experienced by the spouse or a stranger. Following the text, a corresponding happy, sad, or neutral face image of the partner or stranger was displayed on the screen. The text descriptions were included in the empathy protocol to reduce cognitive ambiguity and enhance emotion-specific effects because context plays an important role in emotion processing, as observed by emotion researchers [57, 58]. Following each facial photo display, a large (4-digit) number was shown on the screen. Participants were instructed to mentally count backward by 7 s, starting with a large number, to reduce carryover effects between stimuli. This sequence was repeated so that no 2 conditions followed each other consecutively.
Immediately after the empathy task, while still in the scanner, participants provided emotion ratings of each face image to confirm that the corresponding emotion was evoked. The instructions read, “Now you will see a series of emotion words. Please rate how you felt while viewing images of “X.” Where X was placed either [a] PARTNER SMILING, [b] PARTNER FROWNING, [c] STRANGER SMILING, or [d] STRANGER FROWNING appeared on the screen. A series of positive (e.g., joy) and negative (e.g., sadness) emotion words appeared on the screen. Participants were asked to rate each emotion via a button response box on a scale from 1 (not at all) to 4 (a great deal).
rs fMRI Task
The second part of the scanning protocol consisted of a 5-min rs fMRI scan where participants were instructed to “just relax for the next 5-min,” while a blank screen was displayed. The rs brain scan was the main unit of our analysis, as described in Introduction.
fMRI Acquisition and Analyses
Scanning was conducted at UCSB’s Brain Imaging Center (BIC) using a 3T Siemens Prisma magnetic resonance imaging system with a standard 64-channel head coil. Anatomical data were collected using a T1-weighted magnetization prepared rapid gradient echo sequence (TR = 2,500 ms; TE = 2.22 ms; FA = 7; 208 slices; 0.9 mm3 isotropic voxels). Prior to functional data acquisition, we collected a gradient recall echo field-map (TR = 400 ms; TE1 = 4.92 ms; TE2 = 7.62 ms). All functional scans employed T2*-weighted single-shot gradient echo, echo planar imaging sequences sensitive to the BOLD contrast (TR = 2,000 ms; TE = 30 ms; FA = 90; 3 × 3 mm in-plane resolution; 3 mm slice thickness). Whole-brain coverage was collected in 37 interleaved slices with generalized autocalibrating partially parallel acquisitions (acceleration factor = 2).
Data were preprocessed using Statistical Parametric Mapping 12 software (SPM12, Wellcome Trust Centre for Neuroimaging, London) and several in-house MATLAB scripts. First, the functional time series were motion-corrected and unwarped using a voxel displacement map derived from the field-map scan. The mean functional image was coregistered to the high-resolution anatomical scan, and all images were then spatially normalized to the MNI152 template. A 6-mm full-width at half-maximum isotropic Gaussian kernel was applied to smooth the functional data.
Following this initial preprocessing, we implemented additional denoising steps relevant for rs functional connectivity. First, global signal scaling (median = 1,000) was applied to account for transient fluctuations in signal intensity over space and time. Voxel-wise time series were then linearly detrended. In an effort to mitigate both temporal and spectral motion confounds (e.g., due to spin history effects), we performed wavelet despiking [59, 60] and nuisance signal regression. In the latter, nuisance regressors included the Friston-24 expansion of motion parameters [61], accounting for autoregressive and nonlinear effects of head motion, and 5 physiological noise components estimated from CSF and white matter signal. All nuisance regressors were detrended to match the time series; the residuals of this procedure were then used in subsequent analysis.
Functional Connectivity Estimation
Functional network nodes were defined using the 400-region cortical parcellation by Schaefer et al. [62] and 15 subcortical regions from the Harvard-Oxford atlas (http://www.fmrib.ox.ac.uk/fsl/). We extracted summary time series for each node by taking the first eigenvariate across functional volumes [63]. The nodal time series were then spectrally decomposed using a maximal overlap discrete wavelet transform: low-frequency fluctuations in wavelet scale 2 (∼0.06–0.13 Hz) were selected for functional connectivity estimation. Finally, we estimated the zero-order Pearson correlations between all pairs of regional time series, yielding a 415 × 415 functional association matrix for each participant. These were thresholded using an FDR correction (p < 0.05) to minimize the potential for spurious associations and Fisher-Z transformed prior to group-level analysis.
Statistical Analysis
To assess covariance between HSP Scale scores and functional connectivity, we performed an edgewise regression analysis. All data were mean-centered, and connectivity estimates at each network edge were regressed against each participant’s HSP Scale score. Given the small sample size, we implemented robust empirical significance tests for each analysis using 10,000 iterations of nonparametric permutation testing. In this procedure, the edgewise connectivity estimates were randomly permuted, models were fit, and 2-tailed p values were derived as the proportion of cases in which the absolute value of the permuted test statistics (t-values) was greater than or equal to the absolute value of the “true” estimate. The resulting whole-brain statistical parametric maps were corrected for multiple comparisons using an edgewise FDR threshold (q < 0.05).
In an effort to parse out the direction and magnitude of brain-behavior associations across each functional network, we computed nodal association strengths per graph theory treatment of signed, weighted networks. Here, the thresholded statistical maps were used to derive summary estimates of both positive and negative associations (independently) at each node by summing the positive/negative edges linked to them, respectively. We then assessed the mean strength of association (±95% confidence intervals) in each direction—when applicable—across the constituent nodes of each network (as defined by the Schaefer cortical atlas and the Harvard-Oxford subcortical regions). Additional post hoc, seed-based, whole-brain analyses were performed on ROIs identified from previous fMRI studies of SPS (see Table 1). Results were thresholded at p < 0.05, adopting a FWE for multiple comparisons correction [64]. The ROIs occupied a 15-mm radius minimum. However, for brain stem regions, we used a 3-voxel minimum (rather than a larger number) to detect small regional changes. Anatomic regions were confirmed with the Atlas of the Human Brain [65].
Results
Descriptive Statistics
The mean HSP Scale score for the present sample was 4.09 (SD = 1.26, range = 1.60–5.90). There was an adequate distribution of HSP Scale scores, similar to those found in larger studies of HSP Scale with normative populations [42] and also with the 11-item version of the HSP Scale [53]. N (M = 3.04 ± 1.35) was significantly correlated with SPS (r = 0.77, p < 0.01); thus, we controlled for it in our analyses as in other research using the HSP Scale.
Post-Task Emotion Ratings
Results for the empathy task emotion ratings are shown in Figures 1 and 2. SPS was negatively correlated with calmness in response to partner happy images (r = −0.60, p < 0.05), also with love (r = −0.60, p < 0.05) and compassion (r = −0.62, p < 0.05) in response to stranger happy images. Interestingly, SPS was negatively associated with love (r = −0.64, p < 0.05), compassion (r = −0.65, p < 0.05), joy (r = −0.65, p < 0.05), excitement (r = −0.66, p < 0.05), energy (r = −0.62, p < 0.05), and calmness (r = −0.56, p < 0.05) in response to sad partner images. These results are consistent with prior research showing diminished neural reward activity in association with greater SPS in response to negative images among a sample of adults [37]. No significant effects were shown in response to the stranger sad or neutral conditions.
Post-scan fMRI happy task emotion ratings: Participants reported significantly higher positive emotions in response to partner happy (M = 3.71 ± 0.25) versus stranger happy (M = 1.78 ± 0.60) face images, p < 0.01.
Post-scan fMRI happy task emotion ratings: Participants reported significantly higher positive emotions in response to partner happy (M = 3.71 ± 0.25) versus stranger happy (M = 1.78 ± 0.60) face images, p < 0.01.
Post-scan fMRI sad task emotion ratings: Participants reported significantly higher negative emotions in response to partner sad (M = 2.38 ± 0.21) versus stranger sad (M = 1.52 ± 0.38) face images, p < 0.01.
Post-scan fMRI sad task emotion ratings: Participants reported significantly higher negative emotions in response to partner sad (M = 2.38 ± 0.21) versus stranger sad (M = 1.52 ± 0.38) face images, p < 0.01.
rs Connectivity Analyses
Positive Connectivity Results
Across all networks, participants showed patterns of increased rs brain connectivity as a function of greater SPS (measured with the HSP Scale). Of the 7 networks that we examined, the most robust SPS-related brain connectivity was shown in the ventral attention, dorsal attention, and limbic networks.
As shown in Table 2, ROI (seed) analyses revealed numerous between-network positive correlations as a function of greater SPS. Notably, in the default mode network (DMN), significant positive SPS-related connectivity was shown between the precuneus and the hippocampus (see Fig. 3), and between the insula and the inferior parietal lobe (IPL). In the ventral attention network, significant SPS-related connectivity was shown between the hippocampus and the anterior cingulate cortex (ACC); the precuneus with the dorsolateral prefrontal cortex, posterior cingulate gyrus (CG), insula, and the hippocampus; the medial frontal gyrus (MFG) with the CG, IPL, inferior frontal gyrus (IFG), and the ACC; and the insula with the IPL and premotor cortex.
Increased resting-state functional connectivity seed clusters associated with Sensory Processing Sensitivity
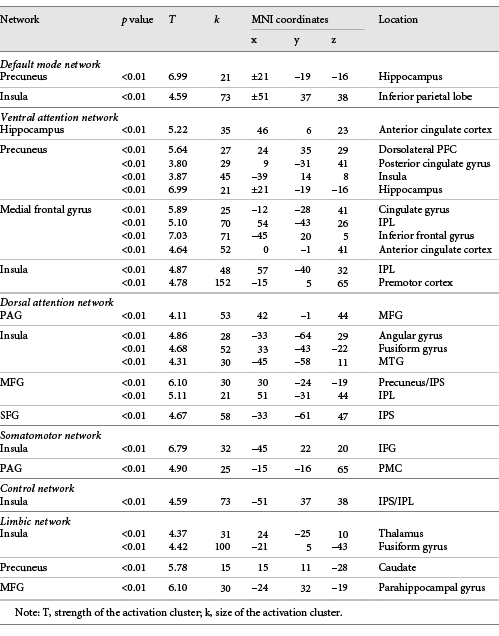
Increased connectivity of the left precuneus with the right hippocampus significantly correlated with higher SPS scores. SPS, sensory processing sensitivity.
Increased connectivity of the left precuneus with the right hippocampus significantly correlated with higher SPS scores. SPS, sensory processing sensitivity.
In the dorsal attention network, SPS was significantly correlated with increased connectivity of the periaqueductal gray (PAG) with the MFG; the insula with the angular gyrus, the fusiform gyrus, and middle temporal gyrus; the MFG with the precuneus/intraparietal sulcus (IPS) and the IPL; and finally, between the SFG with the IPS.
In the somatomotor network, SPS-related connectivity results were shown in the insula (both R and L sides) with the IFG, and the PAG with the premotor cortex (PMC). In the control network, significant SPS-related connectivity was shown between the insula and the IPS/IPL, while the limbic network showed increased connectivity between the left insula and the thalamus, the right insula with the fusiform gyrus, the precuneus with the caudate, and the MFG with the parahippocampal gyrus.
Negative Connectivity Results
Table 3 shows ROI results for SPS-related between-network negative connectivity which corresponds with weaker (or antiphase) rs brain connectivity. In the default mode network, decreased SPS-related rs connectivity was shown between the PAG with the superior temporal sulcus, MFG, and middle temporal gyrus; the insula with the hippocampus; and the hippocampus with the SFG and precuneus.
Decreased resting-state functional connectivity seed clusters associated with Sensory Processing Sensitivity
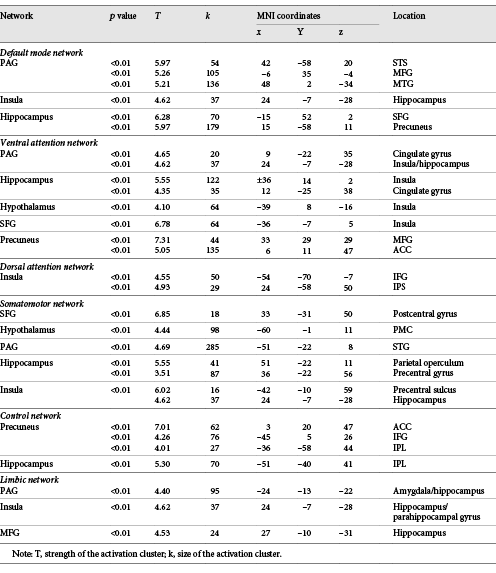
In the ventral attention network, SPS showed the weakest connectivity between the PAG with the CG and the insula/hippocampus; the hippocampus with the insula and CG; the hypothalamus with the insula; the SFG and the insula; and the precuneus with the MFG and ACC. For the dorsal attention network, SPS showed significant decreased connectivity between the insula with the IFG, and the insula with the IPS.
In the somatomotor network, SPS showed decreased connectivity between the SFG and the postcentral gyrus; the hypothalamus and the PMC; the PAG and the STG; the hippocampus with the parietal operculum and precentral gyrus; and the insula with the precentral sulcus. The control network also showed significant negative correlations between the precuneus with the ACC, IFG, and the IPL; and the hippocampus with the IPL.
Finally, regions of the limbic network also showed significant decreased connectivity with SPS, most notably between the PAG with the L amygdala/hippocampus (see Fig. 4); the insula with the R hippocampus (see Fig. 5); and the MFG with the R hippocampus. Overall, the hippocampus, classically known for its role in memory processing, emerged as an important center of both decreased and increased rs connectivity in association with SPS.
Negative connectivity of the right PAG and left amygdala/hippocampus significantly correlated with higher SPS scores. SPS, sensory processing sensitivity; PAG, periaqueductal gray.
Negative connectivity of the right PAG and left amygdala/hippocampus significantly correlated with higher SPS scores. SPS, sensory processing sensitivity; PAG, periaqueductal gray.
Negative connectivity of the right insula and right hippocampus significantly correlated with higher SPS scores. SPS, sensory processing sensitivity.
Negative connectivity of the right insula and right hippocampus significantly correlated with higher SPS scores. SPS, sensory processing sensitivity.
Discussion
SPS is a biologically based trait associated with greater responsivity to environmental and social stimuli. A central feature of SPS is the depth of processing from multisensory modalities. Although depth of processing confers benefits, such as greater empathic ability, attention to detail, creativity, and aesthetic sensibility, there are some costs associated with the trait. For example, highly sensitive individuals tend to experience overstimulation, overarousal, cognitive depletion, and greater need for rest. Although prior studies have investigated the neural basis of SPS in response to emotionally evocative and visual stimuli, to date no study has examined individual differences in rs brain connectivity as a function of SPS. Thus, we examined SPS-related rs brain connectivity among a group of individuals subsequent to their completion of a social affective (“empathy”) task while in the scanner.
Consistent with several theories proposing depth of processing as a signature feature of SPS, the present study revealed stronger connectivity in several clusters reflecting enhanced cognitive features [2, 66, 67]. For example, robust connectivity clusters were observed in regions implicated in higher order cognitive processing, consolidation of memory, reflective thinking, and preparation for action. One key finding showed robust SPS-related rs connectivity between the bilateral hippocampus and the left precuneus (Fig. 3), whose co-activation has been associated with episodic memory and better performance on memory tasks [68‒71]. Increased connectivity of the hippocampus-precuneus has also been associated with dynamic, involuntary memory retrieval that is triggered by naturally occurring perceptual cues, without conscious effort [70]. In contrast, several studies have shown decreased functional connectivity between the precuneus and hippocampus in AD and related dementias [69, 72‒74]. In addition, a gene-related study of SPS found that rs precuneus activity mediated the association between SPS and dopamine-related genes (found to predict the SPS trait) [75]. Consistent with our findings, the dopamine genes had a suppression effect on regional spontaneous activity in the precuneus, which was positively linked to SPS.
These findings indicate that memory processing, specifically episodic memory, is amplified as a function of higher sensitivity (SPS) during “rest” after a social/affective task. The enhanced memory would seem to support depth of processing in SPS and ultimately awareness of, attunement to, and responsiveness to environmental and social stimuli [33]. It has been proposed that improved memory consolidation for emotionally relevant information after a challenging, stressful, or important event is an adaptive strategy which helps organisms prepare for and face similar situations in the future [76]. For example, memory consolidation enables the organism to integrate and utilize information to engage with the environment adaptively and efficiently, and to remember what is rewarding but also what is harmful. These findings are consistent with theories suggesting that SPS may be an adaptive strategy (via enhanced awareness and responsivity) for responding to emerging environmental situations [22, 33], including identifying potential opportunities (such as food, mates, alliances, and suitable environments), and also threats (such as predators, harsh environments, and toxic substances). Some theorists have even suggested that enhanced responsivity to aesthetically pleasing objects, such as landscapes and other positive stimuli, is an adaptation that provided our ancestors with advantages to distinguish suitable from nonsuitable environments [77, 78]. Thus, these findings lend support to the idea that enhanced memory processing is one mechanism by which SPS confers greater depth of processing, which ultimately supports readiness to respond successfully to future events.
It is important to note that the robust memory-related brain connectivity patterns observed in the present study may have been partly due to the nature of the sample that underwent a 6-week mind-body program. For example, prior studies showed improvements in memory in association with the meditation practice (Kirtan Kriya) which our participants practiced for 6 weeks [47, 79]. Also, enhanced memory effects were likely due to SPS characteristics which show enhanced responsiveness to interventions [39, 40].
Other interesting results showed significant negative SPS-associated connectivity within the limbic network, between the amygdala and the PAG (Fig. 4). The amygdala is well established for its role in emotion processing and response to threatening stimuli via fear induction [80]. The PAG, largely known for its role in pain modulation, also mediates coping strategies in response to threat or stress, mainly through projections from limbic regions, such as the amygdala and insula [81, 82]. When threats are detected by the amygdala, the selection of a response is often dependent on its connectiveness with the PAG. The PAG outputs to motor control areas that guide the expression of behavioral responses to facilitate resolution of the threatening event [83]. Thus, the connectivity between the amygdala and the PAG is critical for a variety of behaviors such as harm avoidance, freezing, and prosocial behaviors such as the protection of offspring and altruism [84]. The PAG is also involved in the detection of internal physiological signals that trigger defensive behaviors [85]. As such, the amygdala-PAG circuit modulates states such as anxiety, fear, panic, and pain, and also calm, attachment, and protective behaviors [80, 86].
Research with nonhuman primates also suggests an important role for the PAG in SPS. For example, the PAG metabolism mediates connectivity of the amygdala and the bed nucleus of the stria terminalis. The strength of this connection is thought to be heritable and associated with an anxiety trait that is similar to SPS in primates [87]. Rodent models examining 5-HTTLPR (serotonin) in rats and mice also suggest an important role for the PAG and amygdala in high sensitivity. 5-HTT knockout rodents are used as animal models of SPS as their phenotypes align with sensitivity characteristics such as increased emotionality, decreased exploration of open spaces, and increased response to sucrose reward under threat [5, 88]. Studies have shown that fear-conditioning differentially affects 5-HTT knockout mice and rat neural activity in the motor and (somato) sensory cortices, the insula, and amygdala (increased activity). Also, 5-HTT knockout rats show altered activity of GABAergic neurons that project from the amygdala to the PAG [89]. The present study and animal studies examining the amygdala-PAG link may have clinical relevance for high-SPS persons (which show heightened anxiety in both childhood and adulthood [36]), as well as other populations experiencing high levels of stress and mood-related issues. Thus, connectivity of the amygdala-PAG may be a critical subject for future research.
Other significant findings from the present study showed negative connectivity in the limbic network between the hippocampus and insula as a function of increasing SPS (Fig. 5). The hippocampus and insula are structurally and functionally connected, and their connectivity is associated with emotion processing, stress regulation, and mood disorders [90‒92]. For example, acute social stress was shown to be associated with enhanced brain connectivity of the hippocampus-insula circuit in an experimentally induced social stress task [93]. Another study showed increased connectivity of the insula and hippocampus in association with the processing of untrustworthy faces [94]. Other studies have shown increased connectivity of the insula and hippocampus in adults with stress-related disorders, including depression [95‒97]. Some researchers have suggested that the hippocampus-insula pathway may be co-activated during stressful events to integrate the information into emotional memory [98]. Some researchers speculate that stress favors a habitual, automated response system rather than a slower, cognitively demanding, deliberative system, so that the individual may readily respond to the stressor [99]. However, the habitual response may result in dysfunctional inhibitory control over time, associated with intrusive thoughts or reliving experiences, as observed in clinical disorders such as post-traumatic stress [100]. Thus, these findings may also have clinical relevance.
In contrast, in the present study, we observed decreased rs brain connectivity of the hippocampus and insula, rather than the typical habitual stress-related connectivity pattern. Thus, these findings suggest higher order deliberative cognitive processing in association with SPS. Other evidence of higher order processing was observed as enhanced connectivity of the hippocampus with the precuneus (associated with higher order memory consolidation) and the hippocampus with the ACC (implicated in learned safety in both mice and humans [51]). Collectively, these results support theories suggesting depth of processing as a cardinal feature of SPS [2, 3]. They also highlight one mechanism that supports depth of processing: deliberative cognitive processing during the brain’s rs.
Other evidence of higher order, deliberative cognitive processing associated with greater SPS was shown by enhanced connectivity across several networks, such as the DMN and somatomotor networks. Most notably, in association with SPS the insula showed stronger connectivity with various regions of the DMN (precuneus, IFG, and IPL) and the dlPMC. SPS was also correlated with enhanced connectivity of the caudate (a reward/motor region) with the precuneus, and the parahippocampal gyrus with the MFG, indicative of emotional memory processing. Moreover, the dorsal and ventral attention networks, which are involved in the deployment and reorienting of attention (including automatic alerting to auditory and visual systems), showed the largest widespread connectivity in association with SPS. These results are also consistent with theory and research suggesting depth of processing and awareness as signature features of SPS [2, 3, 33].
Future Directions and Limitations
The present study is the first to examine individual differences in rs brain connectivity as a function of SPS, thus advancing our knowledge of the trait and corresponding rs brain processes. Although this research advances the field, additional research is needed not only because this works suggests other questions, but also because it has its limitations. One clear limitation of this study is the small sample size. However, this limitation was offset by a few factors. One was that the effect sizes reported herein were moderate to large. This was partly due to SPS-related amplification of effects, as the trait is associated with increased responsiveness to interventions. Also, our sample selection was largely constrained to meet the study requirements of fMRI participation and engagement in the 6-week mind-body program. We chose to constrain the selection criteria to optimize recruitment and reduce noise, which helps to enhance neural signals. Also, the thresholds that we applied for the connectivity analyses were conservative, thus limiting false-positives. Last, our a priori ROIs were based on previous fMRI studies, also increasing the power of the analyses. Thus, even with a small sample, relatively large effect sizes were observed.
However, the sample homogeneity (in terms of age, race, education, and socioeconomic status) and its unique nature present an opportunity for future research to address the limitation of the compromised generalizability of the present results. Indeed, this was a unique sample as participants were all caregivers likely experiencing increased stress due to caring for a partner with MCI. However, we might argue that “caregiving” is an essential attachment behavior shown to be critical in the mammalian attachment system [101, 102] and a common life event that many people experience. These factors increase the ecological validity of the study.
Nevertheless, further research is warranted, with larger samples and a wider range of demographics. Research with larger samples and more power would facilitate investigation of genetic polymorphisms that underlie the unique rs brain connectivity patterns conferred by SPS. Studies with larger samples would also allow for more sophisticated modeling, such as determining the direction of correlations. For example, although a negative correlation was observed for the amygdala-PAG circuit, either the amygdala or the PAG might have been showing higher or lower activation. However, there were a few negative correlations (ACC-precuneus and CG-hippocampus) that suggest physiological homeostasis rather than anxiety for our participants. For example, the ACC and precuneus show increased rs brain connectivity in panic disorder [103]. However, in this study we observed a negative correlation consistent with lack of or reduced anxiety, or the presence of homeostatic balance. This interpretation is also consistent with research showing heightened activation of the PAG in response to positive stimuli in association with SPS [37]. Indeed, neuroimaging studies suggest that SPS is associated with heightened reward response to positive stimuli.
Also, it will be important to use a variety of stimuli and experimental protocols to assess the neural connectivity associated with SPS under different conditions. For example, future studies might assess how SPS mediates neural responsivity to auditory, sensory, or olfactory stimuli. Using various stimuli would contribute to more refined results and a broader understanding of the neurobiology of SPS.
Conclusion
This is the first study to report individual differences in rs brain connectivity as a function of SPS. Consistent with theories and research suggesting that depth of processing is a central feature of SPS, the present study showed enhanced connectivity in a network of regions reflecting higher order processing of information, memory, and attention. SPS was positively associated with connectivity of the hippocampus with the precuneus, whose connection is associated with episodic memory consolidation. Further support for depth of processing in the present study was shown by robust negative connectivity of the hippocampus with the insula, which is implicated in higher order, deliberative consolidation of memory, rather than habitual memory processes. Finally, SPS was significantly correlated with connectivity of the amygdala-PAG circuit, suggestive of physiological homeostasis.
In sum, the present findings suggest that when given the opportunity to rest, after engaging in a social/affective task, high (vs. low-)-SPS individuals show enhanced patterns of neural connectivity in regions implicated in deliberative cognitive processing, memory consolidation, and physiological homeostasis. These results are consistent with theory and research proposing that depth of processing is a central feature of SPS. They are also consistent with self-report studies finding that those with high SPS report needing more downtime and rest. Finally, this study revealed some of the important functions that occur during the brain’s resting-state, such as deep cognitive processing and memory consolidation, which are enhanced for those with high SPS.
Acknowledgements
We would like to thank the team of research assistants, Sheerin Zarinafsar, Connie Lan, Harini Pathak, and Pia von Strasser for all their efforts, and the BIC Technician Mario Mendoza for assisting in collecting the brain imaging data.
Statement of Ethics
All data collection and reporting associated with this manuscript were carried out in compliance with internationally accepted standards for research practice and reporting according to the Committee on Publication Ethics (COPE) guidelines.
Conflict of Interest Statement
The authors have no conflicts of interest to declare.
Funding Sources
Funding for this study was provided by the Alzheimer’s Association (AA). The AA was not involved in study design, preparation of the data, or development of the manuscript.
Author Contributions
B.A. was the principal investigator for the study and manuscript write-up. R.M. assisted in data analysis, preparation of figures, and manuscript write-up. T.S. was involved in brain imaging data analysis, methods, and preparation of figures. E.A. and A.A. were involved in manuscript write-up.