Enteroviruses (EVs) have been connected to type 1 diabetes in various studies. The current study evaluates the association between specific EV subtypes and type 1 diabetes by measuring type-specific antibodies against the group B coxsackieviruses (CVBs), which have been linked to diabetes in previous surveys. Altogether, 249 children with newly diagnosed type 1 diabetes and 249 control children matched according to sampling time, sex, age, and country were recruited in Finland, Sweden, England, France, and Greece between 2001 and 2005 (mean age 9 years; 55% male). Antibodies against CVB1 were more frequent among diabetic children than among control children (odds ratio 1.7 [95% CI 1.0–2.9]), whereas other CVB types did not differ between the groups. CVB1-associated risk was not related to HLA genotype, age, or sex. Finnish children had a lower frequency of CVB antibodies than children in other countries. The results support previous studies that suggested an association between CVBs and type 1 diabetes, highlighting the possible role of CVB1 as a diabetogenic virus type.
Introduction
A connection between enterovirus (EV) infections and human type 1 diabetes has been documented in a variety of studies (1–3). Meta-analyses of studies on direct detection of EVs in blood or tissues have indicated a clear risk effect (odds ratios [ORs] 5.5–17.4) (4), whereas serological studies have shown inconsistent results (5). Accordingly, invasive infection, as reflected by the presence of EV in blood or tissues, rather than superficial (i.e., mucosal) infection is possibly needed for the development of β-cell damage.
On the other hand, many serological studies have been based on methods that cannot differentiate EV types from one another. In a scenario where only some of the >100 different EV types would induce type 1 diabetes, their effect could easily be missed if only pan-EV assays are used (antibodies against diabetogenic EV types can be masked by antibodies against all other EV types). For example, one can argue that if the etiology of poliomyelitis would have been evaluated in retrospective case-control studies, it would have been impossible to identify the three EV types that cause polio paralysis (poliovirus types 1–3) with such broadly reactive pan-EV antibody assays because the background frequency of EV infections was high when poliovirus antibody studies were conducted. This aspect is particularly relevant if antibodies are measured a long time after the causative infection, such as at the time when type 1 diabetes becomes clinically apparent.
The current study was designed to detect antibodies specifically against each of the six group B coxsackievirus (CVB) serotypes in patients with type 1 diabetes and matched control subjects. We focused on the CVB group of EVs because previous studies have shown that human pancreatic islets strongly express the coxsackievirus and adenovirus receptor (CAR) (6). CAR is the major receptor for CVBs and is not used by other EV types (7). Because the receptor binding partly explains the tropism of different EV serotypes to various organs (e.g., polioviruses to the central nervous system), the expression of CAR in the pancreatic islets fits with possible tropism of CVB group EVs to these cells. In fact, previous studies have documented the presence of EV RNA and EV proteins in the pancreatic islets of patients with type 1 diabetes (8–10), but the serotype of the involved EVs has not been identified. CVBs have also been linked to diabetes in mouse models, case reports, and case-control studies. In the early studies of the 1960s, Gamble et al. (11) reported neutralizing antibodies against CVB group EVs more frequently in patients affected by type 1 diabetes than in control subjects. Later studies identified species B EV sequences in the blood of type 1 diabetic patients, and CVB4 has been isolated from the pancreata of two patients with newly diagnosed type 1 diabetes (12,13). In addition, we have recently observed that of 41 different EV serotypes screened in prospectively observed children carrying HLA genes conferring susceptibility to type 1 diabetes, only CVB serotypes modulated the risk of β-cell autoimmunity and clinical type 1 diabetes (14).
In the current study, the past exposure to different CVB serotypes was analyzed in children with type 1 diabetes and control subjects recruited in five European countries. The study was based on the measurement of neutralizing antibodies, which are specific and sensitive indicators of past infection by a given EV serotype, thus reflecting the infection history of the child (serological scar).
Research Design and Methods
The study population included 249 patients with newly diagnosed type 1 diabetes and 249 control subjects who were matched pairwise according to the time of sampling, sex, age, and country (Table 1). They were recruited in Finland (103 case-control pairs), Sweden (58 pairs), England (13 pairs), France (43 pairs), and Greece (32 pairs) between 2001 and 2005 in the European Union–funded Viruses in Diabetes (VirDiab) study. In Finland, the patients and control subjects were also matched for the HLA-conferred risk for type 1 diabetes since control subjects were recruited from the Diabetes Prediction and Prevention (DIPP) birth cohort study, which observes children who carry HLA risk genes (15). In other countries, control subjects were healthy schoolchildren or children coming to the local hospital for minor elective surgery. Children with malignant or autoimmune diseases, with chronic infections (e.g., hepatitis), and from infectious disease wards were excluded as control subjects. A case child’s family members or classmates were also not accepted as control subjects. However, children with sporadic and common acute infections were not excluded. The diagnosis of type 1 diabetes was based on World Health Organization criteria. Serum samples were collected for virus antibody analyses shortly after the diagnosis of type 1 diabetes (mean 3 days; range 0–31 days). In the control group, corresponding serum samples were collected an average of 68 days after the diagnosis of type 1 diabetes in the matching case child. Because neutralizing antibodies reflect past infections and their prevalence was not associated with the season of the year, we accepted a long time difference in older children (range 512 days before to 514 days after the corresponding case sample). Blood was collected in EDTA tubes at the same time for genetic studies. Samples were stored at −80°C until analyzed.
Virus Antibodies
Neutralizing antibodies were measured against all six CVB serotypes (American Type Culture Collection [ATCC] prototype strains) with a plaque neutralization assay at the Department of Virology, University of Tampere, Finland. In addition, antibodies against CVB1 and CVB3 serotypes were measured with the use of wild-type virus strains (PicoBank strains isolated in Finland). The serotype of all viruses was confirmed by sequencing the VP1 coding region of the viral genome (16). The serum was first mixed with 100 plaque-forming units of the virus and incubated for 1 h at 37°C followed by overnight incubation at room temperature. This mixture was then transferred to a monolayer of green monkey kidney cells on six-well plates in plaque assay medium containing minimal essential medium supplemented with 1% FBS, 40 U/mL penicillin-streptomycin, 0.0023% glucose, 1 × l-glutamine, 1.5 mmol/L MgCl2, and 1.5 mmol/L carboxymethyl cellulose (HEPES). The number of plaques was counted after 48 h of incubation at 37°C. All test runs included both virus-positive and virus-negative control wells (17). Sera were tested in two dilutions (1/4 and 1/16), and the sample was judged seropositive if either of these dilutions inhibited >80% of the plaques. Samples were also analyzed for higher titers (1/64, 1/256, and 1/1,024) of antibodies against ATCC CVB strains.
Diabetes-Associated Autoantibodies
Islet cell antibody (ICA) was detected by indirect immunofluorescence, whereas insulin autoantibody (IAA), GAD antibody (GADA), and insulinoma-associated protein 2 autoantibody (IA-2A) were quantified with radiolabel binding assays as previously described (18).We used cutoff limits for positivity of 2.5 JDRF units for ICA, 3.48 relative units (RU) for IAA, 5.36 RU for GADA, and 0.43 RU for IA-2A, representing the 99th percentile in >350 Finnish children. The disease sensitivity and specificity of the ICA assay were 100 and 98%, respectively, in the fourth round of the International Workshops on Standardization of the ICA assay. The disease sensitivity of the IAA assay was 58% and the specificity 100% in the 2005 Diabetes Autoantibody Standardization Program Workshop. The same characteristics of the GADA assay were 82 and 96%, respectively, and those of the IA-2A assay were 72 and 100%, respectively.
HLA Genotyping
Alleles in the HLA-DQB1, -DQA1, and -DRB1 genes were defined by panels of sequence-specific oligonucleotides and the presence or lack of disease-associated HLA-DRB1*03-DQA1*05-DQB1*02 and HLA-DRB1*04-DQA1*03-DQB1*03:02 haplotypes documented in each subject (19). Altogether, 189 patients and 171 control subjects were available for genetic analyses, providing 148 HLA-typed case-control pairs.
Statistical Analyses
Descriptive statistics are presented as proportions, frequencies, and means for demographic data and proportions and frequencies for genotypes and autoantibodies. ORs and 95% CIs were estimated for factors associated with type 1 diabetes, using conditional logistic regression to account for the matched-pair study design. Two-tailed P < 0.05 was considered statistically significant. Data were analyzed using Stata 12.1 (StataCorp LP, College Station, TX) statistical software.
Results
Neutralizing Antibodies Against CVBs in Different Countries and Age-Groups
The prevalence of antibodies against different CVB serotypes varied. CVB2, CVB3, CVB4, and CVB5 were the most common serotypes, whereas CVB1 and CVB6 were less frequent (Fig. 1). CVB antibody prevalence also varied considerably among countries. The most striking difference was that Finland had fewer CVB infections than other countries: All six CVB serotypes were most uncommon in Finland except CVB6, which was relatively rare in all countries (Fig. 1). The antibody prevalence increased by age in children <8 years old; thereafter, a plateau was observed (Fig. 2A). Altogether, 28% of the children were seropositive for at least one CVB serotype before the age 4 years compared with >80% of children >8 years (P < 0.001). This age association varied for different CVB serotypes. CVB1 was frequent already at a very young age but did not show as sharp an increase at older ages as did CVB2 to CVB5 (Fig. 2B). The average antibody levels also showed clear variation among CVB types: Antibody responses against CVB3 and CVB4 were the strongest, whereas the antibody responses against CVB1 and CVB6 were the weakest (Fig. 3).
Prevalence of neutralizing antibodies against different CVB serotypes in various countries (neutralizing antibody titer ≥4 by plaque assay). Black bars represent CVB1; horizontally striped bars, CVB2; white dotted bars, CVB3; white bars, CVB4; black dotted bars, CVB5; and skew striped bars, CVB6.
Prevalence of neutralizing antibodies against different CVB serotypes in various countries (neutralizing antibody titer ≥4 by plaque assay). Black bars represent CVB1; horizontally striped bars, CVB2; white dotted bars, CVB3; white bars, CVB4; black dotted bars, CVB5; and skew striped bars, CVB6.
Proportion of children positive for CVB antibodies in different age-groups (neutralizing antibody titer ≥4 by plaque assay). A: Children positive for at least one CVB serotype. B: Children positive for individual CVB serotypes. ◇, children positive for CVB1; ♦, CVB1 wild type; ●, CVB2; ▲, CVB3; △, CVB3 wild type; ○, CVB4; ■, CVB5; □, CVB6.
Proportion of children positive for CVB antibodies in different age-groups (neutralizing antibody titer ≥4 by plaque assay). A: Children positive for at least one CVB serotype. B: Children positive for individual CVB serotypes. ◇, children positive for CVB1; ♦, CVB1 wild type; ●, CVB2; ▲, CVB3; △, CVB3 wild type; ○, CVB4; ■, CVB5; □, CVB6.
Relative proportion of low and high antibody titers among children seropositive for different CVB serotypes. Altogether, 249 patients and 249 control subjects were analyzed for the presence of different titers of antibodies against ATCC CVB strains. Black bars represent a titer of 1/4; skew striped bars, titer 1/16; dotted bars, titer 1/64; horizontally striped bars, titer 1/256; and white bars, titer 1/1,024. Statistical significance when the distribution of CVB1 antibody titers was compared with that of other serotypes are as follows: CVB1 vs. CVB2, P = 0.0001; CVB1 vs. CVB3, P = 0.0001; CVB1 vs. CVB4, P = 0.0001; CVB1 vs. CVB5, P = 0.008; and CVB1 vs. CVB6, P = 0.0001.
Relative proportion of low and high antibody titers among children seropositive for different CVB serotypes. Altogether, 249 patients and 249 control subjects were analyzed for the presence of different titers of antibodies against ATCC CVB strains. Black bars represent a titer of 1/4; skew striped bars, titer 1/16; dotted bars, titer 1/64; horizontally striped bars, titer 1/256; and white bars, titer 1/1,024. Statistical significance when the distribution of CVB1 antibody titers was compared with that of other serotypes are as follows: CVB1 vs. CVB2, P = 0.0001; CVB1 vs. CVB3, P = 0.0001; CVB1 vs. CVB4, P = 0.0001; CVB1 vs. CVB5, P = 0.008; and CVB1 vs. CVB6, P = 0.0001.
Neutralizing Antibodies Against CVBs in Patients With Type 1 Diabetes and Control Subjects
Neutralizing antibodies were first measured against all six CVB types by ATCC CVB prototype strains. Antibodies to CVB1 were the only ones whose prevalence differed between the case and control subjects, being more frequent in diabetic children (Table 2). This finding was confirmed with a wild-type CVB1 strain that gave almost identical results of a significantly higher prevalence of CVB1 antibodies among patients (Table 2). The risk character of CVB1 was seen in both sexes and in different age-groups (data not shown). This risk character was also observed when different antibody levels were used as a cutoff for seropositivity (titer 1/16, OR 1.7 [95% CI 0.9–3.4], P = 0.133; titer 1/64, 1.6 [0.8–3.5], P = 0.198; titer 1/256, 2.0 [0.7–5.9], P = 0.206) and when the effect of CVB1 was adjusted for the effect of the HLA-DQ genotype (adjusted OR for ATCC CVB1 1.6 [0.8–3.3], P = 0.173), although these analyses were not statistically significant. (Only a subgroup of children was analyzed for different antibody levels and HLA alleles.)
Neutralizing antibodies against different CVB serotypes in patients with type 1 diabetes and control subjects
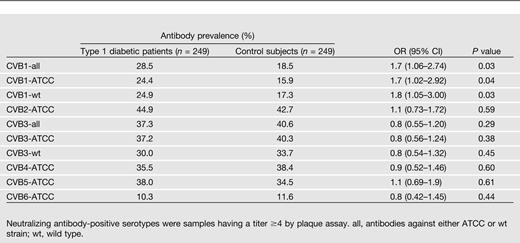
Because the prevalence of CVB antibodies was considerably lower in Finland than in other countries, we compared CVB antibodies between case and control subjects separately in Finland and the other countries. CVB1 showed a similar trend for increased frequency in case subjects both in Finland (OR 2.2 [0.8–5.7]) and in other countries (1.5 [0.8–3.0]), although the difference was not statistically significant in these subgroups.
None of the other CVB serotypes differed between case and control subjects. The overall cumulative number of antibodies to different CVB types also did not differ between these groups (mean 1.8 vs. 1.7, respectively).
Discussion
The results of the current study are in line with those from previous studies, suggesting an association between CVBs and type 1 diabetes. In the current study, CVB1 was the only CVB serotype showing this association. The significance of this finding is emphasized by the very same virus type recently being observed to increase the risk of type 1 diabetes in the large, prospective DIPP study (14). In DIPP, EV infections were identified by measuring neutralizing antibodies against 41 different EV serotypes in children who were followed from birth and who developed multiple type 1 diabetes-associated autoantibodies as well as in control children. The risk association of CVB1 was seen already before type 1 diabetes–associated autoantibodies appeared, suggesting a potential role of CVB1 infections in initiating β-cell damage (14).
The identification of CVB1 as a risk virus in these two independent studies supports the biological significance of this finding because the detection of the same signal in both studies just by chance is unlikely, especially with the study designs being different in many ways. The current study is based on a cross-sectional design that included children who had recently been diagnosed with clinical type 1 diabetes, whereas the DIPP study was based on a prospective birth cohort where samples had been taken during the prediabetes period. In principle, the detection of neutralizing antibodies in the current study covered all past CVB infections, including those that occurred after the β-cell damaging process began. In addition, antibodies were measured against ATCC reference strains, whereas only wild-type strains were used in DIPP; additionally, DIPP samples were analyzed in another laboratory. Of most importance, the current study covered different countries and populations, whereas the DIPP study was carried out only in Finland, and the children analyzed in these two studies were conceived during different time periods. Nonetheless, the risk character of different CVBs still may vary according to time and place, and we cannot exclude the possibility that other CVB types, such as CVB4, could also be associated with type 1 diabetes in a certain time period or geographic region, as suggested in previous studies (11–13).
One limitation of the current study is that the case and control subjects were not completely matched for type 1 diabetes–associated HLA genotypes. Previous studies suggested that these genotypes may modulate antibody responses against EVs (20) and detection of EVs in patients with type 1 diabetes (21). The HLA genotype is also known to modulate the immune response and the course of many other viral infections (22,23). However, such an HLA effect can hardly explain the elevated CVB1 antibodies in patients with type 1 diabetes because the risk effect of CVB1 remained when adjusted for the effect of HLA, and it was seen in Finland where case and control subjects were matched for diabetes-associated HLA genotypes. In addition, the same risk effect of CVB1 was recently observed in the DIPP study, where case and control subjects were matched for type 1 diabetes–associated HLA genotypes (14). An additional limitation of the current study is that the time of sample draw was not exactly the same in patients and their matched control subjects (i.e., the sample from control subjects was taken an average of 68 days after the diagnosis of type 1 diabetes in the case patient). However, considering that neutralizing antibodies remain elevated for years after infection and that the samples were taken randomly all year round and at a later time point in control subjects, the timing of sample draw is an unlikely explanation for the increased frequency of CVB1 antibodies in the case patients.
The measurement of neutralizing antibodies allowed us to study the past exposure to individual CVB serotypes in an unbiased way. These antibodies persist for years or decades and are a sensitive indicator of past infection. They are also highly specific for the EV serotype used in the assay, suggesting that elevated CVB1 antibodies in patients with type 1 diabetes are not elicited by unspecific immune reactivity. The specific nature of this observation is supported by the fact that the difference in CVB1 antibodies between case and control subjects was seen at both low and high antibody levels and that the antibodies against all other CVB types did not differ between subject groups. Furthermore, neutralizing antibodies are biologically active because they neutralize the infectivity of the virus and correlate with immune protection against that EV serotype. On some occasions, however, the neutralizing antibody response can remain low and even be transient, especially if the infection is caused by a low dose of the virus (24). Therefore, the prevalence of neutralizing antibodies in this kind of cross-sectional retrospective survey may underestimate the true number of past infections, making it difficult to assess the proportion of diabetes cases that could be causally linked to CVBs.
On the basis of the current findings, the neutralizing antibody levels against CVB1 and CVB6 were lower than those against other CVB types, and they did not increase by age as clearly as antibodies against other serotypes did, suggesting that a proportion of CVB1 and CVB6 infections may have remained undiagnosed. This kind of variation in neutralizing antibody responses against different serotypes has also been described after vaccinations with live poliovirus (i.e., poliovirus type 1 and 3 induce lower antibody responses than poliovirus type 2). Such a low neutralizing antibody response may also have biological significance, making the virus able to evade the host’s immune response. This is seen clearly in patients with antibody deficiencies who can develop severe and chronic EV infections. This scenario has been proposed for human parechoviruses, which are close relatives of EVs (25).
In our previous study in the prospective DIPP birth cohort, CVB3 was observed to have a strong protective effect against type 1 diabetes (14). Further analyses indicated that this effect could be mediated by immunological cross-protection against CVB1 infections, attenuating its diabetogenic effect. However, this kind of protective effect of CVB3 was not detected in the current study. One possible explanation is the chronological order of CVB1 and CVB3 infections. In the DIPP series, we were able to identify the exact timing of infections by measuring antibodies in longitudinal follow-up samples, and the protective effect of CVB3 was related to early CVB3 infections that occurred before CVB1 (14). Accordingly, the possible protective effect of early CVB3 infections may have remained unrecognized in the current study because this serological scar may have been faded by antibodies induced by CVB3 infection occurring after CVB1 infection.
The prevalence of CVB antibodies was lower in Finland than in other counties, supporting our previous studies that found a relatively low frequency of EV infections in Finland (26). In contrast to EV infections, the incidence of type 1 diabetes is exceptionally high in Finland (the highest in the world at ∼60 per 100,000 children per year) (27). The frequency of EV infections has also declined over the past decades in both Finland and Sweden, whereas the incidence of type 1 diabetes has increased in both countries (17). On the basis of these findings, we launched the polio hypothesis, which claims that a low frequency of EV infections in the background population increases the risk of EV-induced β-cell damage (28). This hypothesis is based on an analogous experience from another EV disease, paralytic poliomyelitis, which is the well-known complication of poliovirus infection and similarly associated with a low frequency of poliovirus infections in the population (28). The polio hypothesis has recently been supported by mouse studies, showing that the absence of maternal EV antibodies increases the risk for severe outcomes of an EV infection in offspring (29). However, in the present study this relationship was not absolute because, for example, Sweden had a relatively high frequency of infections but has a high incidence of type 1 diabetes.
In conclusion, the current study supports the idea that CVBs may include diabetogenic virus types and indicates that CVB1 may be one of them. However, according to the present data, predicting how large a fraction of type 1 diabetes could be causally related to CVB infections is difficult. Experience from other EV diseases, such as polio, suggests that a very small proportion of infected individuals will ever develop the disease, leading to a scenario where the antibody prevalence can be almost similar in case and control subjects in this kind of cross-sectional study (30). Therefore, prospective studies are needed to confirm the risk effect of CVB infections and to evaluate their etiologic fraction. In any case, the present observations together with previous studies showing that CVB1 infection is frequent (31) and able to cause insulitis and islet cell damage in young babies (32) as well as pancreatitis, transient diabetes (33,34), and persisting infection in mice (2) make it an attractive target for further studies addressing the possible role of EVs in the pathogenesis of type 1 diabetes.
P.M. is currently affiliated with the Public Health Laboratory Bristol, Public Health England, Bristol, U.K.
Article Information
Acknowledgments. The authors thank Anne Karjalainen, Mervi Kekäläinen, and Eveliina Jalonen, University of Tampere, for technical assistance.
VirDiab Study Group: Heikki Hyöty, Sami Oikarinen, and Kaisa Kankaanpää (University of Tampere); Didier Hober, Bernadette Lucas, Jacques Weill, Chantal Stuckens, and Guy-André Loeuille (University Lille 2 and CHRU Lille and CH Dunkerque); Peter Muir (King's College London); Keith Taylor (Queen Mary and Westfield College); Glyn Stanway and Cigdem Williams (University of Essex, Colchester); Christos S. Bartsocas, Andriani Vazeou, Evangelos Bozas, Maria Dokopoulou, and Dimitris Delis (National University of Athens); Johnny Ludvigsson (Linköping University); Päivi Keskinen and Marja-Terttu Saha (Tampere University Hospital); Jorma Ilonen (University of Turku); and Mikael Knip (University of Helsinki).
Funding. This study was supported by competitive research funding of the Tampere University Hospital, the JDRF, the Academy of Finland, the Päivikki and Sakari Sohlberg Foundation, and the European Commission (VirDiab Project, Quality of Life Programme, KA2, contract number QLK 2-CT-2001-01910 and PEVNET FP-7 Programme, contract number 261441).
Duality of Interest. H.Hy. and M.K. are both minor (<5%) shareholders and members of the board of Vactech Ltd., which develops vaccines against picornaviruses. No other potential conflicts of interest relevant to this article were reported.
Author Contributions. The members of the VirDiab Study Group participated in the planning of the study. S.O. organized the laboratory work and data analyses, wrote the manuscript, and reviewed and edited the manuscript. S.T. and A.S.-K. reviewed and edited the manuscript, organized the virus antibody analyses, and participated in the data analysis. D.H., B.L., A.V., E.B., P.M., H.Ho., G.S., C.S.B., and J.L. reviewed and edited the manuscript. J.I. reviewed and edited the manuscript and was responsible for the HLA genotyping. M.K. reviewed and edited the manuscript and was responsible for the autoantibody analyses. P.K. and M.-T.S. reviewed and edited the manuscript and were responsible for the recruitment of study subjects in Finland. H.Hu. reviewed and edited the manuscript and carried out the statistical analysis. K.T. reviewed and edited the manuscript and was responsible for the recruitment of study subjects in England. H.Hy. was the coordinator of the VirDiab Project and responsible for the overall scientific management of the project. S.O. is the guarantor of this work and, as such, had full access to all the data in the study and takes responsibility for the integrity of the data and the accuracy of the data analysis.
Prior Presentation. Parts of this study were presented in poster form at the 17th meeting, EUROPIC 2012, Saint-Raphaël, France, 3–7 June 2012.